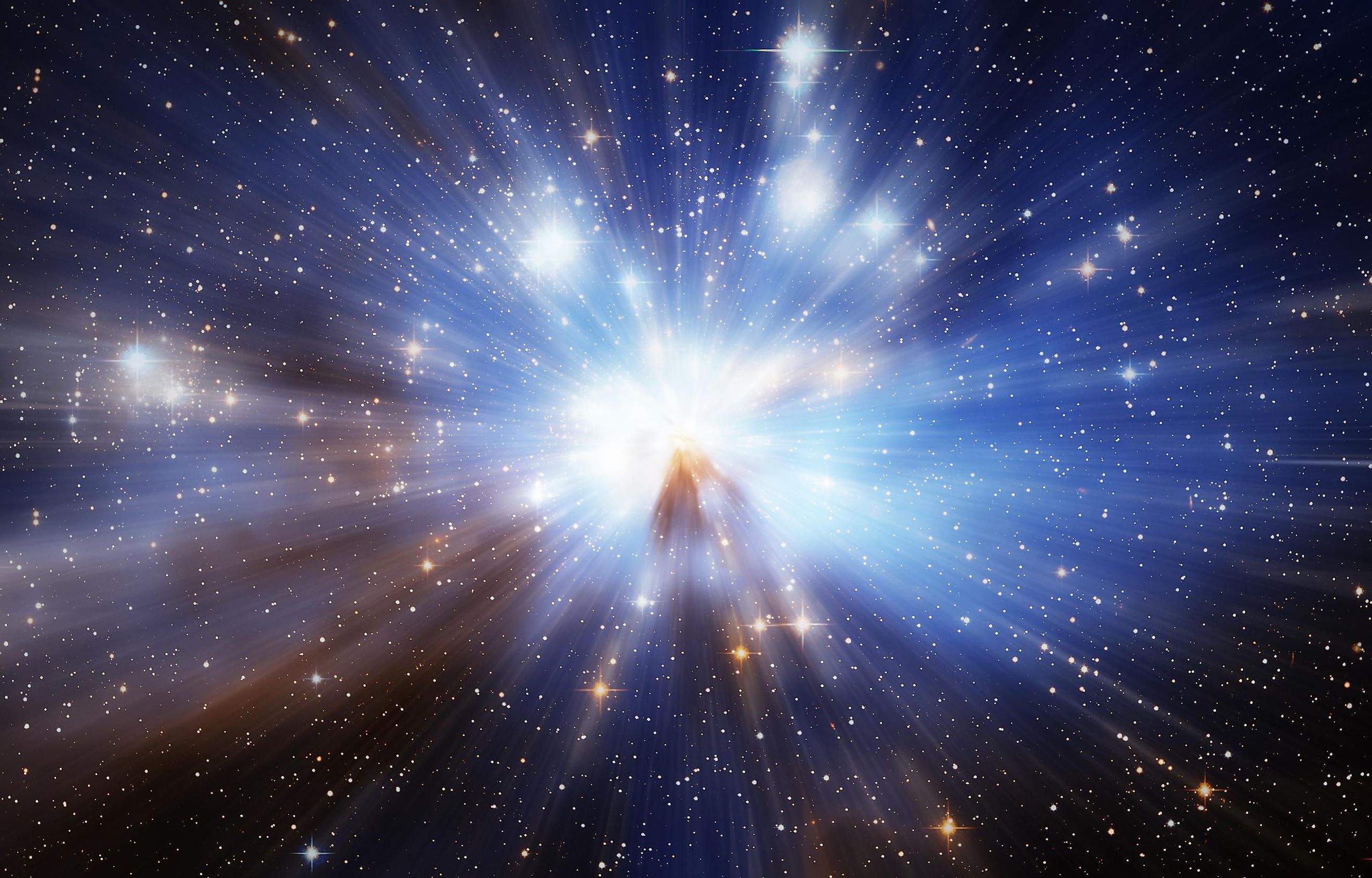
What Was The Big Bang?
For as long as humans have existed, we have strived to understand how the world around us came to be. Every culture and civilization throughout history has developed their own version of how the universe came into existence and evolved. With the development of modern science in the 20th century, research has revealed that the universe likely began 13.8 billion years ago in an event called the Big Bang. What was the Big Bang and how was it discovered?
Steady State vs the Big Bang
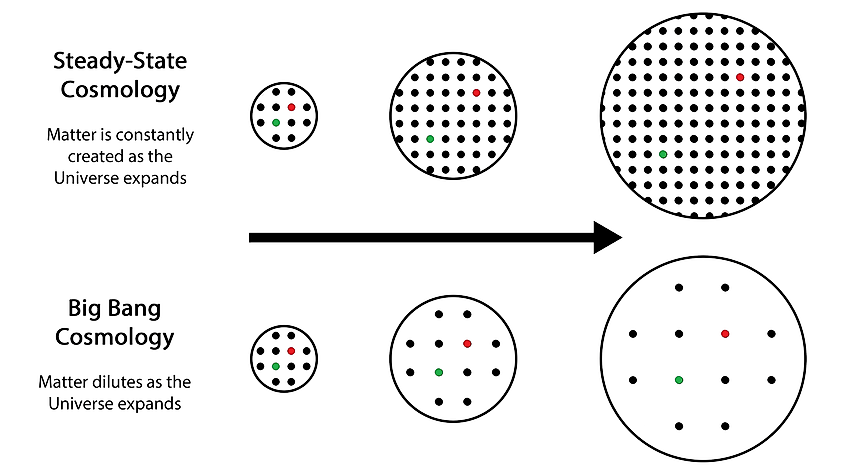
Although the Big Bang model of the universe is nearly universally accepted among scientists, it was not always this way. Prior to the 1950s and 60s, a large group of physicists adhered to what was known as the steady-state theory. The steady-state theory can be thought of as the opposite of the Big Bang. Rather than having the universe begin a finite time ago in a high density, high temperature state, the steady-state theory claimed the universe was infinite in age, and that everywhere in the universe was identical to every other location. The steady-state theory was first proposed in the 13th century and it held sway until the mid-20th century. The first major challenge of the steady-state theory arose in 1929, when the astronomer Edwin Hubble published his discovery that the universe is expanding. Hubble’s discovery challenged the steady-state model in that it showed that the universe changes over time, something the steady-state model did not predict. However, the steady-state was modified to predict that the universe continually creates matter as the universe expands, allowing it to exist in the same state for an infinite amount of time. Interestingly, even Albert Einstein believed in the steady-state, even modifying some of his equations that predicted a finite universe in order to agree with a static, eternal universe.
Starting in the 1920s, some scientists began proposing theories that the universe had a finite age and was in fact a dynamic, ever-changing place. This was in direct contrast to the steady-state theory. Then, in 1931, physicist Georges Lemaître proposed that if the universe is expanding, then the universe must have been smaller in the past. He stated that if time were run in reverse, the entire universe would have occupied a single point in space a finite time ago. This idea soon became known as the Big Bang, and a debate soon began between proponents of the Big Bang theory and the steady-state theory.
The Cosmic Microwave Background Radiation
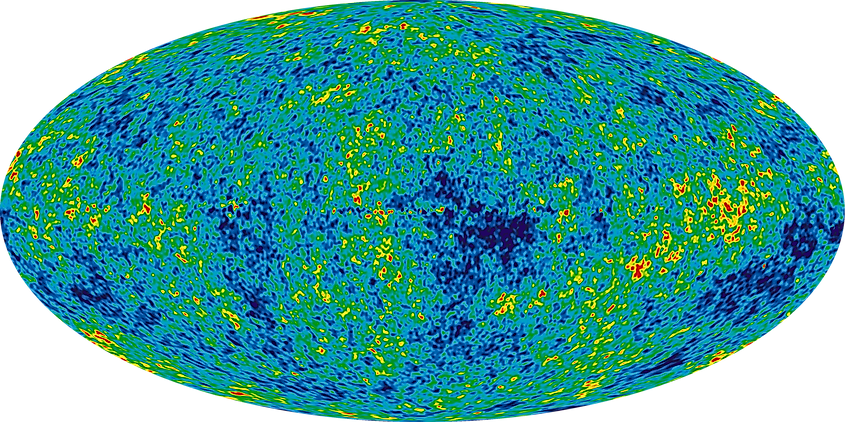
In order for an idea to become a scientific theory, it must be able to make predictions that can be proven through either observation or experiment. In the case of the Big Bang theory, it predicted that the early universe would be saturated with a kind of afterglow; radiation left over from the Big Bang itself. The steady-state model predicted the exact opposite, that since the universe is eternal and unchanging, no such afterglow would exist. In 1964, Arno Penzias and Robert Wilson discovered a source of microwave radiation that they could not account for. Further analysis revealed that they had in fact discovered the afterglow radiation of the Big Bang, called the Cosmic Microwave Background Radiation (CMBR). Analysis of the CMBR offered strong evidence that the Big Bang theory was correct, and the steady-state model was soon disproven and rejected by most scientists.
Timeline of the Big Bang
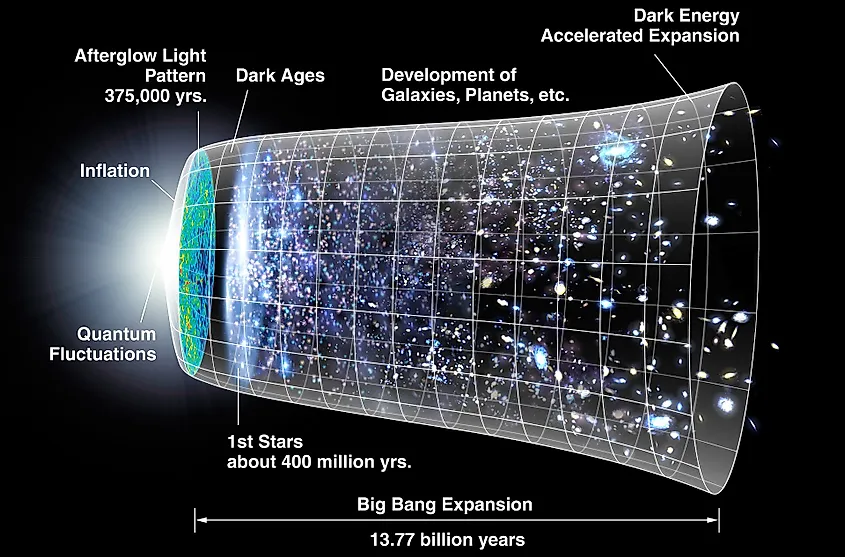
The initial state of the universe 13.8 billion years ago is known as a gravitational singularity; a point of infinite density and temperature that existed a finite time ago. In a way, the term “singularity” can be thought of as a fancy way of simply saying “I don’t know.” A singularity is simply a point where conditions become so extreme that the known laws of physics break down and do not work. The very first moment of the Big Bang is thus shrouded in mystery, and all known laws of physics are unable to explain what was going on when the universe was a singularity.
The first moments of the Big Bang are currently poorly understood as there is no way of directly observing what happened. Rather, scientists rely on mathematical models and experiments in particle accelerators to determine what may have happened immediately after the Big Bang. It is important to note that the Big Bang unfolded over an extremely small scale of time, so small that conventional units like seconds and milliseconds simply do not work. For example, the Plank Epoch, which directly followed the singularity, occurred 10^-43 seconds after the Big Bang. That’s a decimal followed by 43 zeros and then a 1, a mind-boggling tiny number. During the Plank Epoch, all of the forces of the universe were unified as a single force. The Plank Epoch ended and the Grand Unification Epoch began, which saw the initial singular force break apart, with gravity separating first. After the Grand Unification Epoch, the universe underwent a phase of rapid expansion called cosmic inflation, wherein the universe expanded many trillions upon trillions of times larger than its original size. This rapid expansion caused temperatures to cool faster than they otherwise would, and cosmic inflation also prevented the universe from collapsing back in on itself. As temperatures began to cool after inflation, energy began to condense into the first forms of matter such as quarks. Less than one second after the Big Bang, the first protons and neutrons came into existence.
Antimatter
The formation of matter also saw the formation of antimatter in near equal amounts. When matter and antimatter meet, they annihilate each other in a blast of energy. Less than one second after the Big Bang, the fate of the universe was in the balance as matter and antimatter began destroying each other. For reasons that remain unknown, the universe produced slightly more matter than antimatter, and so eventually regular matter was all that remained.
Aftermath
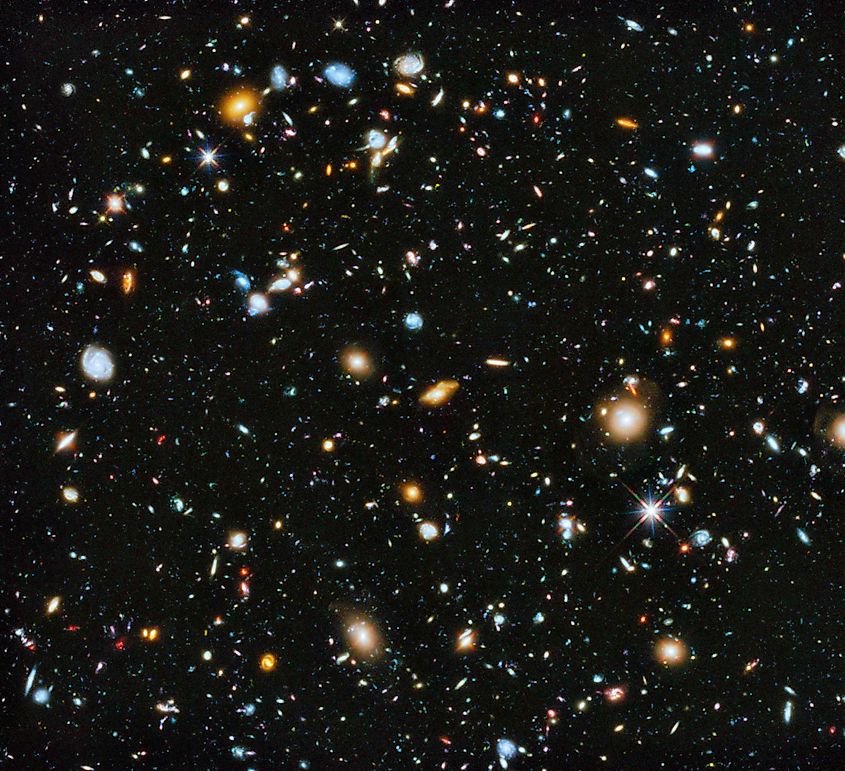
After the first second of time, the stage was set for the universe we now inhabit. Matter had formed, space was expanding, and the four forces of physics were separated. For the next 380,000 years, the universe would be saturated by a dense fog of material, so dense that not even light could traverse the cosmos. However, temperatures eventually began to cool enough that protons and neutrons could capture free electrons, resulting in the formation of the first atoms. The formation of the first atoms caused the dense fog to become transparent to light, and photons could now freely traverse the young universe. Interestingly, it is this light that formed the CMBR that we can observe today.